Figure 1:
Top: A view from near the Salt Lake City cemetery toward the
southwest. KA7OEI's LED is marked by the bright red dot toward the left
of the picture just above the sea of lights - Photo by K7RJ.
Middle: A tightly-zoomed view from KA7OEI's location
toward the the Salt Lake City cemetery. The LED is the obvious
red light in the middle.
Bottom: A wide-angle time exposure view of the above
scene with the foreground vegetation being lit up by the Luxeon and the
background lit by the nearly full moon. The far-end LED is
clearly visible in the middle of the picture.
Click on an image for a full-sized version.

|

|

|
Initial testing:
On Monday, March 19, I dragged my
Optical
Transceiver lens assembly into the field for the first
time: Having only tested it over very short distances, I wanted
to get a "feel" of how bright its beam might be when cast across the
Salt Lake valley. On that evening, at about 9:30, I had arranged
for several people across the valley to look toward my location to give
a report as to what they could see and to judge the brightness of the
beam.
At this point, there was just one optical ("lightbeam") transceiver
available, so
there was no expectation of being able to make a contact: The
only goal was to be seen. I chose a location along the western
edge of the Salt Lake valley along Utah Highway 111 (even with 70th
south) - a location that is higher in elevation than the existing
population centers with no nearby lights. Gordon, K7HFV, was
viewing from a location along the north edge of the Salt Lake City
cemetery: The cemetery was a good choice as it was devoid of
light sources - and because it is close to the mountain, there are few
lights "behind" it. The distance? 14.85 miles (23.9 km.)
Initially, Gordon tried to spot my light from a different location, in
amongst city lights and other buildings. Being that it was now
dark and familiar daylight visual references were now lost, he wasn't
quite sure where to look - and I wasn't sure either. Despite
waving a laser around, hoping that he would catch a glimpse, he saw
nothing, so he went off to find another, darker location.
In the meantime, three other hams were looking for my signal from
another part of the valley. One of them, living in an area with
fewer streetlights, make himself visible by shining a powerful
flashlight in my direction, giving me a precise idea as to where to aim
the optical transceiver. Before too long, he could easily see the
red Luxeon glow. After a few minutes, I swung the transceiver
toward two other hams that lived only a few blocks apart and soon,
they, too were able to see the red glow.
By this time, Gordon found the cemetery location - but I had only a
general idea as to where that was, and he still wasn't sure where I
was. Line-of-sight visibility was soon verified by my shining the
laser around in that general direction, providing a visual reference
for Gordon. After a few minutes, he, too, could see the red
Luxeon glow: He reported that the light was quite bright in
comparison with the other city lights and quite distinctive, owing to
the fact that it was red.
Over the course of the test, it was noted by those that were observing
that the amount of scintillation visibly increased as time goes
on. Owing to geography (see
figure 3 below)
the Salt Lake Valley rests in a bowl. This bowl inevitably
results in a "
temperature
inversion" - that is, the bottom part of the valley is cooler than
the air above. On this particular night, this inversion inversion
was very mild, but was being disturbed by ever-increasing diurnal winds
that flow between Salt Lake valley and Utah Valley (immediately to the
south) and, in fact, these winds were increasing as time went on.
As it turns out, our optical paths were within the boundary layer,
resulting in more disturbance than would otherwise be noted.
Encouraged by these results and getting a better "feel" as to what sort
of visual magnitude could be expected from the focused 3 watt Luxeon, I
decided to construct another
enclosure. While I have onhand two larger, high-quality Fresnel
lenses, I decided to try an experiment and build a cheaper version
using some Fresnel "page magnifier" lenses - the result being shown on
the
"Cheap
Enclosure" web page.
A slight snag:
While I had little doubt that there was enough signal to make a good
contact, I decided to perform a minor modification of the receivers
used in each enclosure to improve the signal-noise ratio: The
installation of a narrowband red
filter in front of the detector to reduce effects of urban
lighting.
Some months ago, I had gone to a local supplier of
theatrical equipment and noticed
that they had some "swatch" booklets containing a small (1"x2") sample
of
each of their theatrical gels along with a handy chart showing the
spectral
response of each. After perusing the "swatch" book and doing some
tests
using a red Luxeon and the booklet's samples, I settled on a
particular gel that I thought looked promising, as it seemed to
attenuation the desired light by only about 1dB.
Several days prior to the test, I happened to be at this same supplier
and obtained a sheet of the color filter gel that I'd considered -
namely
the Roscolux "Fire" (#19) - and paid $6.95 for a 20"x24"
sheet. This filter material (
a graph showing the optical
response versus wavelength of this gel may
be seen here)
looked as though it would be capable of attenuating a signification
portion of the stray light from the urban lighting such as the sodium
D-line emission at about 590nm (although low-pressure
sodium vapor lamps are uncommon) in addition to the strong peak around
559nm, as well as the strong Mercury lines at 579, 546, and 436nm -
leaving mostly the broader emissions that fell within the filter's
passband.
(Note that the photodiode is increasingly
less-sensitive at shorter wavelengths, anyway.)
Out
of this sheet, I cut two pieces (about 1-1/2" or 4cm square) to put in
front of each detector. Because these filters are dye-based, they
are not particularly sensitive to the incident angle of light -
unlike
the more-expensive dielectric filters - so they may be installed at
about
any point in the optical path.
The first test with this gel was done using the "cheap" enclosure - the
one made
of the "foam core" posterboard. Because of the receiver is a
bare
circuit board, I simply taped the piece of color filter gel
to the shields that surround the photodiode on two sides and
then re-installed it back into the enclosure. Much to my
surprise, the entire receiver and
enclosure became
extremely microphonic and the amplified
speaker could not be placed anywhere near the enclosure and it was
quite sensitive to room noises in general: In other words, it
made a pretty good microphone!
"Hmmm..." I thought. I hadn't noticed this problem before, so I
removed
the
gel and added some dabs of silicone to some of the dead-bug wired
components on the receiver assuming that I'd just missed the problem
and assuming that the components' movement might be part of the
problem, reinstalled the gel, and tried it
again: No change - still microphonic.
At this point it occurred to me that the
gel had something to do with it: Removing the gel completely
restored the operation of the receiver to its previous state - that is,
it was only
slightly
microphonic. Clearly, the
vibration of the thin gel was somehow modulating the space around the
photodiode: I surmised that because of the relatively large area
of the
photodiode and the extremely high impedances involved, any slight
changes in the nature of that space (such as the slight change in the
free-space capacitance) would be translated as audio output.
The interim solution was to form a small cardboard enclosure around the
circuit board and use this to space the gel 2 inches or so above the
photodiode. While this configuration greatly reduced the
microphonic tendencies to the point where it was "tolerable" it was
still noticeably more microphonic than
it
had been without the gel. The optical receiver for the
other
enclosure was mounted in a shielded case but its
microphonics were still noticeably worse after installing the
gel:
There, too, I used a piece of cardboard to stand the gel away from the
photodiode an extra inch or to.
It wasn't until the day after the testing that I finally figured out
what had happened: I hadn't thought much about it during the
test, but neither Ron, Gordon or I noticed any problems with
microphonics and the enclosures. Even with the modification on
the "cheap" enclosure, it was still likely that feedback would have
resulted if the amplified speaker had been turned up very loud.
Figure 2:
Top: The equipment used by Ron and Gordon. The
unused septa is in the foreground while the optical transceiver (made
from foam core posterboard) sits behind it on a stone wall bordering
the cemetery - note the headstone on the right.
Bottom: The equipment used at the KA7OEI end. In
the foreground on the low tripod is Ron's laser while the optical
transceiver is sitting on a portable table. Note that with
different fields of view and exposure settings, it is difficult to
judge the absolute positions and brightnesses of the light sources
using these pictures.
Click on either image for a larger version.
 |

|
It finally dawned on me that the mere handling of the filter gel
probably induced a strong electrostatic charge on its surface, causing
it to act exactly like an
electret
microphone. Over the few days between the
installation of the gel and the test, this charge had apparently
dissipated, greatly reducing or eliminating this effect.
The gear that I used:
I used the heavier wooden
Optical
Enclosure
along with its higher quality rigid Fresnel lenses. It has
a
3-watt Luxeon emitter with a
current
limiter with a high quality glass
PCX (PlanoConveX) secondary lens. The optical receiver is the
"Version
3" non-feedback optical receiver (operating from a single 9
volt battery) mounted in a completely
shielded metal enclosure.
The modulator that I used was a
prototype
PIC-based PWM circuit that I
had built in 2006, but had only recently gotten around to
installing in a box. This modulator holds the peak current
constant,
but it varies the duty cycle of the 20 kHz driving waveform to effect
modulation of brightness. In this circuit, the input audio is
amplified, lowpass filtered (for alias prevention) and then applied to
the A/D input of
the PIC that performs (adjustable) audio compression and limiting to
minimize the peak-to-average ratio as well as to prevent
overmodulation. In addition to audio processing, the PIC can also
generate different types of tones that can be used for identification
and peaking of the receive signal as well as for analysis of
scintillation. One feature of this modulator is the ability to
continually adjust LED current from full output all the way down to
just a few milliamps
while still maintaining 100% modulation. This modulator also has
a monitor jack (for use with headphones or audio recorder) to verify
operation of the
modulator, a built-in microphone, a connector for an external
microphone, and a line-in jack for an external audio source such as a
computer or digital audio player.
For listening, I used a homebrew LM386-based powered speaker. The
optical receiver also has a second output that can be fed to a second
audio device, such as a recorder or computer.
On my end I also
used
two digital audio recorders: An inexpensive ($30) 1GB MP3 player
with
a built-in microphone was placed on the table with the gear and used to
capture ambient audio while a more expensive record-capable MP3 player
(with line input)
was connected directly to the optical detector in parallel with the
speaker amplifier's input: Next time, I'll split the audio
channels of
this
unit so that one will be my transmitted audio and the other will be the
received audio.
The gear used by Ron and Gordon:
The Fresnel lens assembly (the
"Cheap
Enclosure") that Ron, K7RJ and Gordon, K7HFV were using had
been
assembled by me (with a little help from Gordon) in just one
evening. This enclosure uses some very
inexpensive "page magnifier" Fresnel lenses that are of fairly low
optical quality mounted in 8"x10" picture frames, with the entire box
being constructed of "foam core" flat black posterboard. While
I'd
also constructed
a
septa from scraps of the foam-core board in the event that
the nearby lights would be a
problem with the rather poor quality of the Fresnel sheet protector, it
turned out not to be required and was never used: It might be
interesting to try it later to see how much reduction of unwanted light
(as well as the signal) results with its use.
In this enclosure I had installed a
3-watt
red Luxeon on a heat sink along with cheap plastic 20mm diameter
DCX (Double ConveX) secondary lens and an
LM317-based
current limiter
to protect the LED in the event of a circuit glitch or
malfunction. Also installed was the original prototype of the
"Version
3" non-feedback optical receiver - a circuit identical to
the final version except that it does not have the 3.5 kHz lowpass
filter or the high/low gain switch. Even though the optical
receiver did not have a lowpass
filter, there were no problems with its reception of the PWM signal
being emitted from my transmitter.
Immediately after constructing the "cheap" enclosure I built a new,
current-linear
modulator based on a design that I'd been kicking around in
my
head: It uses a precision current sink to modulate the LED's
current and allows 100% modulation at any desired resting current
level. In addition to this, it has a built-in audio compressor to
prevent exceeding 100% negative modulation as well as to maximize the
peak-to-average ratio of the audio (to enhance intelligibility) as well
as a PIC-based audio tone generator that can be used for identification
and peaking of the received signal as well as analysis of the amount of
scintillation.
For listening, an inexpensive Radio Shack LM386-based amplified speaker
was used while the microphone was either the unit built into the
modulator or an external computer desk-type or headset-type microphone
- whatever was handy at the time. Some recordings were made at
this end using a cassette tape recorder with an open microphone.
A real, 2-way test:
Figure 3:
Top: Map showing the locations of the two sites and
the path of the link.
Bottom: Vertical elevation profile of the path between
the two sites: Ron and Gordon's site is on the left. While
the air was clear on this evening, additional turbulence resulted from
the presence of a slight temperature inversion - and our optical path
skimmed along the top of the boundary layer.
Click on either image for a larger version.
 |
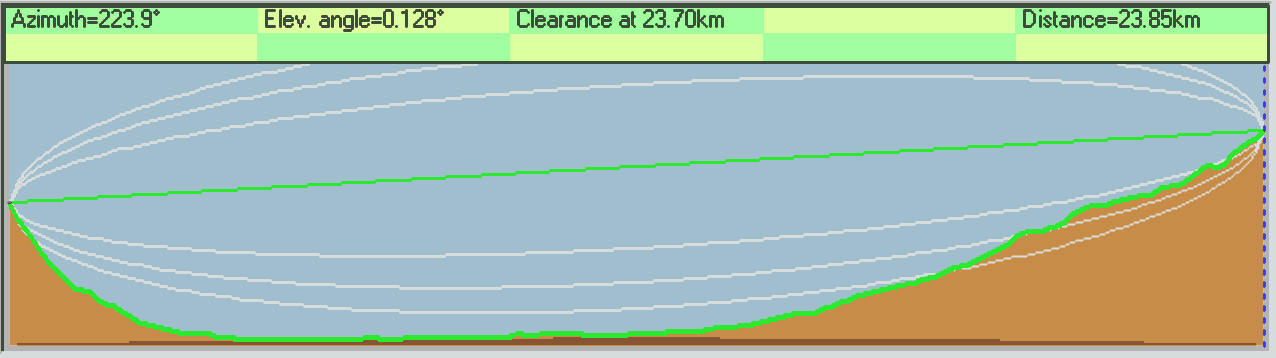
|
On the night of Saturday, March 31, 2007, after meeting with
Gordon, and Ron and his wife for
dinner, I went to a location along Utah Highway 111 very
near where I had been on the 19th (a hundred meters or so north, with
more level ground) with my optical transceiver, while Gordon, K7HFV and
Ron,
K7RJ went back to the Cemetery location that Gordon had scouted out the
week before - this time armed with the
"Cheap
enclosure" and a newly-constructed LED modulator: The
distance? 14.91 miles (23.85 km.) The starting time?
A bit after 9PM and after local dark.
At Ron and Gordon's end, they parked along a road that paralleled the
northern boundary of the Salt Lake City cemetery at an elevation of
about 4860 feet (1480 meters) above sea level. Conveniently,
there was a stone wall demarcating the cemetery's northern boundary and
this appeared to provide a convenient, stable platform for the "Cheap
Enclosure."
On my end, I simply pulled a few feet off the highway into a patch of
dirt
at the crest of a hill, providing a wide panorama of the Salt Lake
valley at an elevation of about 5180 feet (1580 meters) above sea
level. At this location, the ground was slightly muddy from
rainfall a few days past - and this turned out to be pretty
convenient. Setting up a portable table with the car between me
and road, began to pile equipment onto the table and connecting the
gear while Ron and Gordon were doing a similar thing on their end.
After a few minutes, using a 2 meter liaison frequency, I began shining
a laser in their direction so that they would have a solid visual
reference as to my position to facilitate aiming. Powering up my
equipment, I kept an eye open in their direction and it wasn't too long
before I spotted flashes of the tell-tale red Luxeon glow as they swung
their beam past my location. Using 2 meters, I
talked them into a reasonably good aim and then proceeded to point my
transceiver in their direction. After only a few moments, they
could see my LED and after a few more moments of peaking (and pushing
the leg of the table into the mud to fine-tune the elevation setting) I
was shining a reasonably bright light in their direction. After a
quick re-peaking on their end, using the amplitude of a test tone that
I was sending, they were properly aimed toward me. Fortunately,
the careful use of alignment targets and laser pointers
(as
described here)
resulted in the peaking of my signal on their receiver being precisely
coincident with the brightest signal being transmitted by them.
Audio file: This is a stereo audio file, with the left
channel being from a nearby microphone while the right
channel is a recording directly from the optical receiver:
Signal quality:
The general quality of the signals was excellent. As can be seen
from the pictures in
Figure 1, it would
seem that Ron and Gordon fared slightly better in this department
owing to the fact that I was located in an area that was mostly above
all of the city lights. From my vantage point, however, their
light was very near those of downtown Salt Lake City and there seemed
to be a strong, periodic source of 120 Hz buzzing that had a period of
a minute or two: When this source came on, it was quite annoying,
although it didn't affect intelligibility of the transmission from Ron
and Gordon very much. Presuming that the source of this periodic
interference was likely a failing streetlight that was constantly
restriking, I looked - but could never spot it.
Because I had made direct-coupled audio recordings onto a digital
recorder (48kHz sample rate with 16 bit audio) I could measure relative
signal levels and the amount of scintillation present on test
tones.
This 14.91 mile (23.85 km) optical path is directly over a densely
populated metropolitan area, crossing a bowl-shaped valley with a
maximum ground clearance of about 740 feet (225 meters.) Because
of
diurnal breezes the "heat island" effect, and the expected effects of
pollution, scintillation is probably worse than it would be were a
mountaintop-to-mountaintop path used.
Figure 4:
A sample of an audio test tone (932.33 Hz, or A#) that exhibits
the worst-case scintillation noted using the LED and larger Fresnel
lenses.
Click on image for a larger version.

|
A note about the lenses used:
- The "cheap" enclosure being used by Ron and Gordon had identical
Fresnel lenses on receive and transmit that were about 7.25" by 10.25"
(184x260mm) and have a focal length of
approximately 12.25" (about 310mm) and are extremely thin, flexible
(and inexpensive) vinyl "page magnifier" lenses with fairly high line
pitch (about 0.2mm) with rather poor optical
quality.
- The optics on my end were a pair of identical lenses that are
approximately 12-1/2" x 9-7/8" (318mm
x 250mm) with a 13" (330mm) focal length. These are rigid,
molded
lenses that are about 2mm thick and are of good optical quality with
rather coarser (0.5mm) line pitch.
Upon analyzing the audio files, I could see that the amount of
Scintillation varied, peaking at around 17dB, but was typically
less than this. As can be seen from the time scale, the shortest
period of scintillation was typically 23-27 milliseconds (25ms or 40 Hz
nominal) but analysis of other segments of tone indicate that another
component with half this period (11-13 ms or 80 Hz nominal)
would often appear at a much lower amplitude: This shorter period
is only slightly apparent
in
Figure 4. Typically, longer scintillatory periods were
present that were clear multiples of the 25 millisecond period:
This period of scintillation remained fairly consistent throughout the
test period.
Testing with lasers:
Several months ago, Ron had built a pair of laser pointer
communicators: These were modulated (at around 40 kHz) using PWM
techniques and built on some plug-in prototyping board having been
quickly thrown
together for the local radio club's "Homebrew night." Being that
the detectors already being used for the LEDs were compatible with
these lasers, it
was a simple matter of aiming them once we had already aligned the
LED-based
transceivers.
As it turned out that while we knew that aiming with lasers would be
tricky, we had no idea exactly how tricky it would be: Many long
minutes were spent on 2 meters (or over the LED transmitter) saying
things like "weak flash... nothing... nothing... bright
flash..." while each of us
attempted to aim our lasers at each other. On my end,I was
fortunate to be able to plant a tumbleweed into the mud some distance
in front of the laser, providing a handy visual reference to get me
within the ballpark: Fine-tuning was done with great finesse to
finally peak the signal at the other end, relying on the party at the
far end for feedback (e.g. "dim... nothing... Bright
-
Don't touch that!") Ron had a bit more problem pointing his end
owing to the lack of a convenient twig or branch to serve as a
"sighting stick" but was eventually able to align the laser on several
occasions.
There was another problem: Although the laser transceivers were
identical, the one Ron was using had had developed a problem in that it
would manage about 5% modulation, but above this it would distort
terribly. The transceiver on my end started to suffer from a
similar problem, but the installation of a fresh 9 volt battery solved
it - but there was some initial confusion as to why my transmitted
(laser) audio was
still somewhat distorted. In testing
locally, I determined that the modulator on my end was, in fact,
working properly, but that it was the extreme scintillation that was
causing the audio distortion. The scintillation was severe enough
that even the unmodulated carrier sounded "hissy" as the following two
clips demonstrate.
Audio files: These are stereo audio files, with the left
channel being from a nearby microphone while the right
channel is a recording directly from the optical receiver:
- Dead carrier
with laser - This audio clip is the sound of the unmodulated
laser signal: The "hissing" noise of the scintillation is clearly
audible. (MP3, Time = 17 seconds, 346 kB)
- Modulated
carrier with laser - This audio clip includes Ron's voice, but
due to technical problems, the modulation depth was only about
5%. (MP3, Time = 7 seconds, 138 kB)
Figure 5: Examples of
scintillation of the laser signal.
Top: Scintillation of the 440 Hz fundamental frequency.
Bottom: The effects of Scintillation on the 7th harmonic
of the test tone at 3080 Hz. The higher temporal resolution of this
test tone reveals more about the true magnitude of the scintillation.
Note that these are not from the
same portion of the test tone recording - see text.
Click on either image for a larger version.

|

|
Fortunately, the modulator that I was using
did work properly
and it was possible to get some recordings and audio of some test tones
via the laser path. I extracted from Gordon's recording a segment
containing audio of the laser being modulated with a 440 Hz tone.
Upon review of this recording, I could see that my tone generator was
badly overdriving Ron's laser modulator and generating harmonics - but
this turned out to be a
good thing.
In looking at the top image of
Figure 5 you can see the
lowpass-filtered 440 Hz waveform. In this segment one can see
significant scintillation - but only on the order of 15dB or so:
Judging by ear, the the scintillation was far worse than this. In
looking at the unfiltered waveform, I could also see that the period of
the scintillation was fast enough that the 440 Hz fundamental frequency
simply wasn't high enough to show it in detail.
The bottom image of
Figure 5 shows the 7th harmonic (at about
3080 Hz) of the 440 Hz test tone. With the higher temporal
resolution, the true magnitude of scintillation starts to become
apparent: Close-up investigation of the waveform
(using a
tighter "zoom" of smaller portions of this clip) indicates that
there is at least 40dB of scintillation present.
Close-up inspection of the 3080 Hz waveform reveals something
else: Not only is the same 12 millisecond (plus subharmonics)
period present, but there is evidence of harmonics of this period as
well. Unfortunately, the magnitude of these scintillation
harmonics is difficult to discern owing to modulation by the original
440 Hz fundamental frequency of the test tone: In the future,
more tests will be done with a higher frequency audio tone. It
was also interesting to note that when I examined the same segment of
the audio recording shown in the bottom image of
Figure
5 that had been lowpass filtered, only about 10dB of scintillation
was apparent, the true magnitude
being masked by the limited bandwidth.
Audio files: These are monaural recordings of the
signals from KA7OEI sent to Ron and Gordon via laser. The two
clips with the tone contain the segments shown in Figure 5.
Note
that due to MP3 audio compression, the finer
details of the scintillation are lost.
- Lowpass filtered
test tone - This is the recording of the 440 Hz fundamental
frequency, lowpass filtered at about 800 Hz. (MP3, Time = 38
seconds, 224 kB)
- Test
tone - 7th harmonic - The source is the same as the above clip
except that this is the 7th harmonic of the 440 Hz test tone.
With the greater temporal resolution, the true magnitude of the
scintillation becomes apparent. This tone was highpass filtered
at about 2700 Hz. (MP3, Time = 38 seconds, 224 kB)
- Voice recording -
This recording shows the effects of the scintillation on the
voice. The distortion on the signal is believed to be due mostly
to the scintillation, as local testing of the laser's modulation did
not reveal anywhere near this level of distortion. After the
voice ID, there are a few seconds of unmodulated carrier: The
noise you hear is due solely to scintillation. (MP3, Time = 5
seconds, 34 kB)
Comments about the laser tests:
- It should be pointed out that this is not
really a completely fair comparison to demonstrate the amount of
scintillation of a laser's coherent light verses the noncoherent light
from an LED: The transmitted aperture of
the laser was only a few millimeters across, while the
aperture of the LED was over 7"x10" in size - around 10000 times
larger. A much better (and fairer) comparison would be a test
with equal-sized emitter apertures, and this is something that I hope
to do, eventually. (Note: Later tests were
done to provide a fairer comparison of coherent versus noncoherent
light, see the "Our Second
Optical Test" web page.
- It was noted that the brightness of the LEDs were comparable to
the apparent brightness of the lasers, but it was very clear that the
lasers "flickered" far more than the LED, almost seeming
to go out at times.
- For a better measure of scintillation when using this sort of
laser configuration, a fairly high audio tone is required to obtain
sufficient temporal resolution to be able to being to discern the true
magnitude: I hope to do this on a later test. Later
analysis of scintillation was done using a 4kHz tone, as noted on the
web page about the later testing - see above.
Other experiments:
Attenuation test:
Prior to the testing, I did not know what I should expect: Would
the city lights overload the detector? How much margin would I
have? In order to answer these (and other) questions (and to be
prepared in
case of overload) I made an "optical attenuator" which was a
piece of press board with a lot of holes drilled into it in a grid
pattern. This method of attenuation was chosen mostly because I
could not find a piece of dark plastic that I could be sure to block
the vast majority of the incoming light - especially at Infrared
wavelengths where most filters are not rated, but the photodiode is
sensitive.
The obvious way around this was to do what cameras do: Iris
attenuation. It was also important that the aperture of the
Fresnel Lens be maintained (to minimize scintillation) despite the
attenuation, thus the need for the grid of holes rather than one large
hole in the middle of the attenuator.
Audio file: This is a stereo audio file, with the left
channel being from a nearby microphone while the right
channel is a recording directly from the optical receiver:
- Optical
attenuator test - This audio clip demonstrates the effect of
the attenuator on the signal. Partway through the test the gain
on the receiver was switch to "high" as noted in the audio
commentary. (MP3, 59 seconds, 1154kB)
Upon analysis of the file, it was apparent that the attenuator reduced
the signal level by about 30dB. Even with this amount of
attenuation, voice communications with Ron was still possible.
Note
that this test was done before I re-aimed the transceiver and
got another 10dB or so of signal. Also note that this test was
done with the red theatrical gels installed as optical bandpass
filters, and these likely caused a dB or so of additional
attenuation: These gels would not be used in a situation
where
no urban light pollution was anticipated.
Careful aiming is important!
While this test ran for about 3 hours, it wasn't until a bit after the
2nd hour
(and after the attenuator test) that it
finally occurred to me that I hadn't really checked
the peaking of my optical transceiver: At the time of initial
acquisition, I'd simply aimed my LED via feedback from Ron and Gordon
and when they reported seeing it brightly, I left it alone. They
then
used my generated tone to peak their transceiver - but I never
re-checked mine. Up to this point, I was being annoyed by a
fairly
loud racket caused (apparently) by a constantly-cycling street lamp
somewhere in the distance, but after re-peaking the signal, I not only
gained another 10dB of signal from Ron and Gordon, but I moved away
from that annoying noise source, improving the signal-noise ratio
considerably.
Also important - proper focusing and alignment!
Another thing that I noted was that my transmitter and receiver weren't
aimed precisely parallel. After getting home, I determined that
this
was due to the fact that the receiver mounting tube isn't as secure as
it should be: For proper receive focus, I had to pull the tube
out
most of the way and because of this, it would not seat securely and
could wobble about, causing the aiming to shift slightly. This
problem
particularly annoyed me because I was aware of it and even made a new
receiver mount, but had never gotten around to installing and adjusting
it...
A couple of weeks after the test, I constructed a "Photon Beacon" (a
4060 timer/oscillator with a 500 kHz ceramic resonator and a red LED)
and, over a distance of just over 500 feet (about 160 meters) I was
able to precisely adjust the receiver focus and aiming and discovered
that it had been significantly misadjusted. Proper focusing
resulted not only in a gain improvement of at least 10-15dB, but it
also improved rejection of off-axis signals. No doubt I would
have had much better signal margins had the receiver been properly
focused at the time of testing.
I later happened to re-check the receiver focus of the "cheap"
enclosure and found that it was within 1dB of optimum, so I didn't do
anything other than put more thermoset glue on the box to make
everything more stable. I also compared the luminous flux of the
"cheap" enclosure with the wooden enclosure and found that it (the
"cheap" enclosure) was about 4.2dB lower output. Further testing
showed that for receive, the cheap, vinyl Fresnel lenses are
significantly inferior in off-axis rejection of other light
sources: While the beamwidth of the "peak" response is similar to
that of the acrylic Fresnel lenses used in the wooden enclosure, they
produce a somewhat "fuzzy" spot - some of which extends several degrees
in other directions. Additionally, the somewhat inferior lens
material - plus the finer "groove" pitch tends to cause a bit more
random light scattering than the more coarse lines on the acrylic
lenses in the wooden enclosure.
LED current tests:
After re-peaking, we decided to do some tests to see how much we could
reduce the current and still maintain communications. Because both
modulators are designed to allow continuously variable current
adjustment while still maintaining 100% modulation (based on the
resting current) this was a simple matter of turning a potentiometer -
and the presence of a test point on the front panel of the modulator
made current measurement a simple matter of measuring a voltage in
which 1 volt equaled 1 amp.
Note: 1 amp of average
current equates to 2 amps of peak current at 100% modulation.)
Audio file: This is a stereo audio file, with the left
channel being from a nearby microphone while the right
channel is a recording directly from the optical receiver:
- LED Current
Reduction tests - This audio clip demonstrates the reduction of
LED current versus the effects on the signal. Note that partway
through the first segment I increased the gain of the audio clip by
20dB (as evidenced by the sudden increase in noise) to compensate for
the reduction in far-end audio - something that I accounted for at the
time by turning up the volume control on the speaker monitor.
This clip has also been edited to remove "dead spaces" between
adjustments. (MP3, Time = 4 minutes and 45 seconds, 5578kB)
As can be heard from the audio recordings, voice communications under
these conditions was still possible when the current was reduced from 1
amp to 50 milliamps
(a 40dB decrease, assuming a linear
relationship between current and luminous flux - and this is
approximately true) and it was still possible to copy tones via ear
down to 5 milliamps
(a 46dB decrease.) Using narrowband
digital techniques, experiments by others indicate that a further
20-40dB of enhancement is still possible. This in-field contact
confirmed another thing that had been suspected based on testing inside
the house: It is still possible to copy a signal by ear that is
too dim to be seen with the naked eye!
As evidenced by the audio clips, one of the major limiting factors was
the light pollution present: If there had been a sudden power
failure
that had blacked out the entire valley, I have no doubt that we could
have done better that we did as there would have been no 120Hz-related
noise, plus there probably would have been some reduction in "hiss"
from
the myriad of light sources present.
Miscellaneous observations:
- All of us noted all sorts of weird noises that could be
heard when pointing the enclosure at different points around the city
ranging from the 120 Hz roar of the power grid (and its harmonics) to
odd squeaks, grunts, and clicks. Throughout much of the evening I
noted an occasional rhythmic, weak "pop" in the audio that sounded very
much like the strobe of a tower beacon - but I could not see anything
that seemed to correlate with it.
- In previous testing, I noted that I could simply point the
transceiver straight into the air and hear myself via Rayleigh
scattering and reflection from airborne dust - a fact
(carefully)
verified by covering the transmit Fresnel
lens and noting that it disappeared, also indicating that this effect
wasn't due to leakage between the transmit and receive sides of the
enclosure. Testing on a moonless night
(after my eyes adjusted to the dark) I could see the beam projecting
away from the lens into the distance. During the entire test, I
could hear myself (albeit weakly) via this scattering in my own
receiver, but since the signal from Ron and Gordon was so strong, the
gain on the audio amplifier was low enough that feedback was not a
problem. I noted with some amusement that, as large trucks would
pass alongside where I was parked and stir up a cloud of dust, my
"sidetone" audio would suddenly increase - and then fade back again as
the dust settled. Gordon noted that they could hear themselves
via scattering at first, but when they tried it again later, they could
not. Hmmm...
- Despite the use of the low-quality "page magnifier" lens, the
"cheap" optical transceiver seemed to perform about as well as the one
using the "good" Fresnel lens. Of course, side-by-side tests
would be required to do a direct comparison of performance.
- As mentioned before, about 2 weeks after the test, I had the
opportunity to re-check the focus on the receiver that I used and found
it to be far from optimal: Re-focusing resulted in a 10-15dB
improvement in signal gain and better rejection of off-axis
signals. Had the focus been properly adjusted at the time of the
test, I would have experienced significantly better signals.
Final comments and observations:
Audio file: This is a stereo audio file, with the left
channel being from a nearby microphone while the right
channel is a recording directly from the optical receiver:
- Final Comments
- This clip has the closing comments just as we were shutting off our
gear and getting ready to go home. (MP3, 56 seconds, 1108kB)
We finished these test sometime after midnight
(yes, we realized
the implications of the date...) having attracted surprisingly
little attention from the authorities or passers-by. These tests
weren't intended to break any records, but rather give us a "feel" as
to what can be expected.
Also, these "armchair" field trials were intended to reveal problems
with our equipment as well as give experience with the techniques to
align, operate, and optimize it. In no particular order, here are
a few things that we learned:
- Stable platform - This was mostly a problem with the
"cheap" enclosure. Unfortunately, I neglected to make the bottom
flat - something that could have been easily done simply by attaching a
few extra scraps of foam core board. This led to a tendency for
the enclosure to "rock" back and forth on the stone wall and be
extremely difficult to keep it aimed. I didn't have
nearly as much trouble as azimuth adjustment on my end was simply a
matter of rotating the enclosure while elevation was adjusted simply by
pushing the table legs into the mud. Had there not been mud, I
was
still prepared: I had brought two telephone books that could have
been used as adjustable shims, simply by selecting the number of pages
to achieve the desired elevation. Ultimately, some means of
providing a lightweight tripod-type mount (light enough to be carried
up a mountain, but strong enough to be stable) needs to
be devised in case a table or flat, stable rock is not readily
available.
- Fine-tuning adjustments - Despite the use of
"adjustable mud" I still need to construct a "micrometer-type" of
elevation adjustment for my optical transceiver. As can be seen
from the web
page that describes the enclosure, the lid folds underneath - and
it is to the lid that I would attach the elevation adjustment. It
should go without saying that having had any sort of
fine-tuning adjustment in azimuth and elevation would have been of
tremendous help when aligning the lasers! For later testing,
addition equipment was constructed and the enclosure modified to
provide an easy means of fine-tuning the elevation.
- On/Off tone keying - While the modulators have
built-in tone generators, it is clear that I need to add a means of
using a straight key to allow easy sending of CW: Under poorer
conditions, CW may be copyable while voice is not. (It should go
without saying that weak-signal computer modes would do better still...)
- Audio metering - While the tone is useful in peaking
the signal, "better-than-ear" accuracy may be obtained by using an
audio meter of some sort. The caveat is that the inevitable
scintillation is likely to complicate matters, making the resulting
reading bounce around quite a bit, so it is likely that some sort of
longer-term averaging will be required.
- Audio recording interface - Perhaps combined with
the
audio metering should be an interface that mitigates the effects of the
wildly differing audio levels that one can expect in the field.
This device would likely have both a manual gain control and a
slow-acting AGC (as well as a peak limiter) that would prevent
longer-term changes in audio level from either overdriving the recorder
or being too low to be usable. This recording interface would
also provide a means of recording both the transmitted and received
audio to the separate (stereo) audio channels as well as allowing
loopback
to permit the distant party to hear themselves via the entire circuit.
- More laser testing - As mentioned before, it would
be
interesting to do more testing with lasers - especially with the Laser
using the same lens aperture size as the LED. Of course, this
testing would include recordings of tones to measure
scintillation.
Notes about the audio files:
- The left channel was recorded using an inexpensive ($30) MP3
player with a built-in microphone. It was placed on the table
near the optical transceiver and simply allowed to run for several
hours. Because of the open microphone, it picked up not only my
voice, but also the audio from Ron and Gordon's and via the speaker
(when I wasn't using headphones) as well as the sounds of passing
traffic. Inevitably, the sampling rate of this device was
slightly different from that of the recorder used for the other
channel, so there is some evidence of delay (a slight echo or
"hollowness") in some of the tracks.
- The right channel was from a much higher-quality digital audio
recorder connected directly to the audio output of
the optical receiver, so it did not normally contain my transmissions,
except via acoustic coupling from an open microphone on Ron and
Gordon's end and subsequent
retransmission. Another source of audio was from the slight
return due to reflection in the
nearby air from the scattering.
- These audio clips are edited somewhat to remove long spaces of
"nothing" and, in some cases, the gain was adjusted as noted to make
them more listenable. Also, some of the 120 Hz hum (and its
harmonics) was filtered out to avoid overloading the listeners'
speakers and amplifiers.
- The recordings supplied by Gordon were originally recorded to
cassette tape using acoustic coupling.
Return
to the KA7OEI Optical communications Index page.
If you have questions or comments concerning the contents
of this
page, feel free to contact me using the information at this URL.
Keywords:
Lightbeam communications, light
beam, lightbeam,
laser beam, modulated light, optical communications, through-the-air
optical
communications, FSO communications, Free-Space Optical communications,
LED communications, laser communications, LED, laser, light-emitting
diode, lens, fresnel, fresnel lens, photodiode, photomultiplier, PMT,
phototransistor, laser tube, laser diode, high power LED, luxeon,
cree, phlatlight, lumileds, modulator, detector
This page and contents copyright
2007. Last update: 20070725